SNO+555
Overview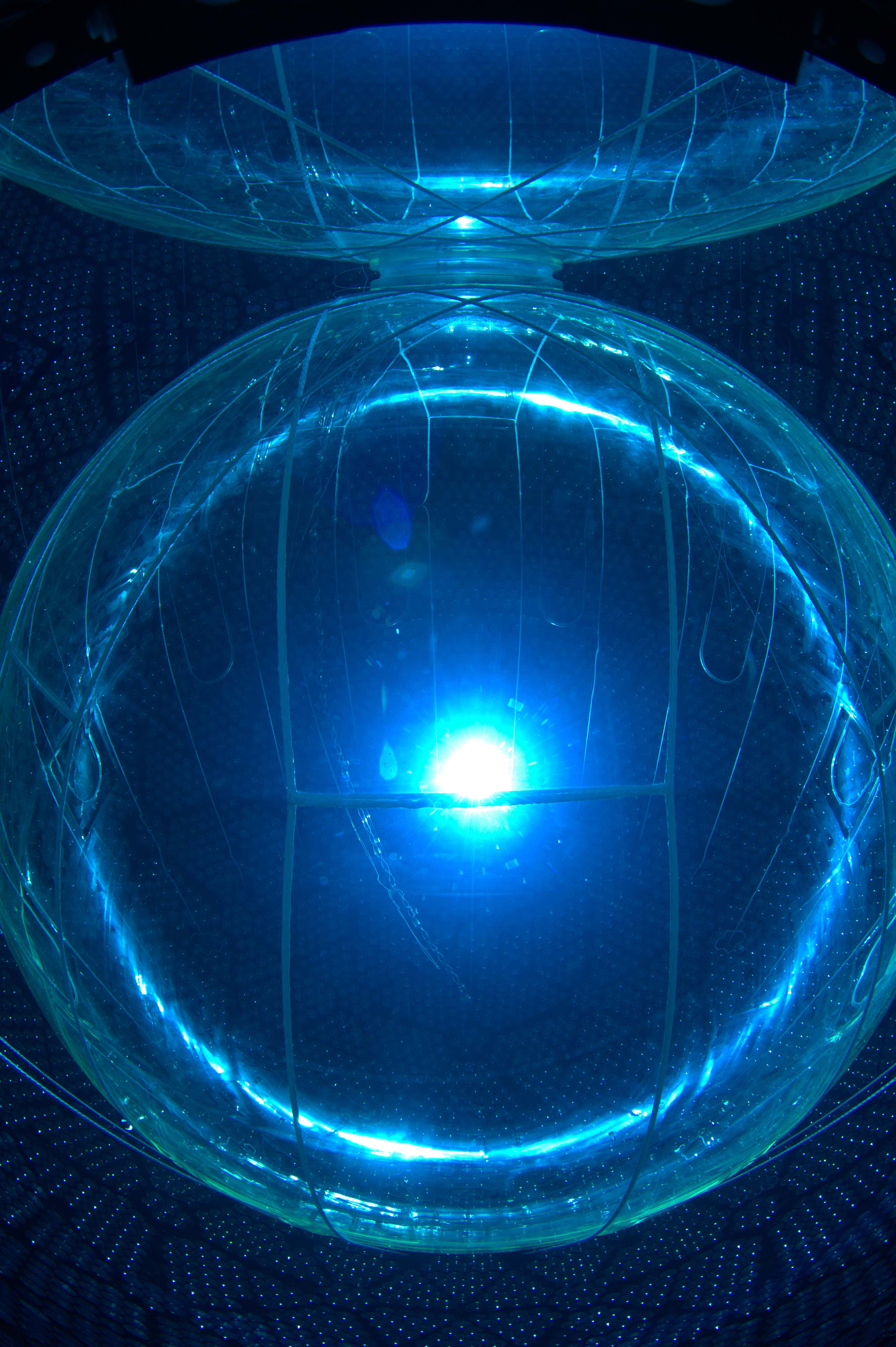
SNO+ is a multiphase experiment with the end goal of looking for neutrinoless double beta decay with 130Te. The experiment is an extension of the original SNO (Sudbury
Neutrino Observatory) experiment, replacing the heavy water from SNO with liquid scintillator doped with 130Te. SNO+ will run in three distinct phases which each allows for the study of different physics topics. The first is a pure water phase which will be sensitive to a unique mode of baryon number violating nucleon decay. The second is a pure scintillator phase which will be sensitive to solar and neutrino oscillation physics, as well as provide an excellent measurement of the detector systematic uncertainties and backgrounds. The final phase centers on the search for neutrinoless double beta decay by adding the 130Te.
The picture shown on the right is a side view taken with an underwater camera during the water phase filling. One can see the Acrylic Vessel hanging from the upper deck, and the surrounding 10,000 8-inch PMTs.
The Search Baryon Number Violation
Baryon number is a symmetry that essentially says that quarks and leptons do not have interactions that change one into the other directly. For example, a proton (baryon number = 1) will not spntaneously decay into an e+ and π 0 (baryon number = 0). Since theories that unify quarks and leptons usually have such interactions, there are theoretical motivations to look for rare decays like this.
SNO+ is uniquely sensitive to the baryon number violating reaction n->3ν that has been suggested as a consequence of low-scale gravity and Large Extra Dimensions (LED). These theories postulate that the fundamental energy scale of gravty is not MPlanck but rather is quite small (M*), and the reason that gravity is so weak is due to propagation in folded up "extra dimensions".
The way to look for this interaction in water is to use the shell structure of oxygen, as shown in the picture on the right (for protons, but neutrons work the same way). A neutron in the 1P3/2 state will cause a 1P1/2 neutron to drop down and emit a 6.18 MeV gamma ray about 44% of the time [5]. The SNO experiment looked for this, but unfortunately neutron capture on D2O also produces a 6 MeV gamma. This resulted in a background limited limit of 2x1029 years for this reaction. KamLAND also looked for this, but since there are no 1P1/2 neutrons in carbon, they were only sensitive to decays from the 1S state, which had to be tagged by an unlikely triple coincidence: (1) 1S state hole de-excitation via fast neutron emission and scatter, (2) beta decay of the daughter nucleus, and (3) neutron capture. Thsi resulted in s very low efficiency and a signal limited limit (there was one candidate event) of 5.8x1029 years.
For the SNO+ water phase, we have oxygen nuclei without the pesky background from D2O, rejection of reactor events via neutron tagging, and solar neutrino electron scattering background reduction via directional imaging. Thus, even in a short water phase (3-6 month) we expect to significantly improve on the KamLAND result. Stay tuned for a result in 2018!
Measurement of Mixing Parameters using Nuclear Reactors
Nuclear reactors used for power around the world are major emitters of electron antineutrinos. These electron antineutrinos are weakly interacting and mostly pass through matter undetected. However, large detectors filled with proton-dense materials can detect these antineutrinos through the inverse beta decay (IBD) process. In an IBD interaction, an electron neutrino hits a proton, producing a neutron and a positron. A double coincidence signal, produced by light emitted from the positron and neutron’s interactions with SNO+’s organic scintillator, can be easily detected using the PMTs.
Measurements of neutrino oscillation parameters have been made through observation of reactor antineutrinos (KamLAND[1]) and solar neutrinos (SNO[2], Super Kamiokande[3]). Upgraded electronics and better calibration/analysis techniques have recently improved solar neutrino oscillation measurements made by the Super Kamiokande detector. The Super Kamiokande collaboration measured the delta-m squared oscillation parameter, defined as the difference of the first neutrino mass squared and second neutrino mass squared. The results indicate a ~2 sigma difference between solar and reactor experimental measurements of the delta-m squared oscillation parameter.
The SNO+ detector will be sensitive to reactor antineutrinos and will make another measurement on the delta-m squared
oscillation parameter[4]. SNO+ is located within 350 km of several Canadian power plants (as shown below).
Due to the location of these power plants, SNO+’s antineutrino spectrum shape is sensitive to changes in the oscillation parameters. We will analyze the antineutrino spectrum shape in SNO+ to provide more data on the present tension in solar and reactor neutrino oscillation measurements. The plot on the right shows the expected antineutrino energy spectrum in SNO+ per MeV for 1032 proton-years of exposure for reactors at the Bruce complex at 240 km (blue), Darlington and Pickering complexes at 350 km (red), and all other reactors (yellow). The grey lines show the unoscillated spectra, plus the expected geoneutrino contribution. SNO+ expects roughly 90 events/year, and thus will be able to make a measurment of Δm212 to a sensitivity of 0.2x10-5 eV2 in about 7 years. This is similar to KamLAND sensitivity, which will help to resolve the current tension between the solar and reactor results.
The Search for Neutrinoless Double Beta Decay
One of the most interesting questions in particle physics is whether or not neutrinos are fundamentally different than other particles because they have a so-called "Majorana Solution." In this scenario, neutrinos are basically their own anti-particle and it is possible that they could mediate neutrinoless double beta decay.
The left side of the figure on the right shows an example of a second order weak double beta decay. Essentially two neutrons decay into protons at the same time, giving two electrons and two electron anti-neutrinos in the final state. This is expected from the
Standard Model and has been seen very clearly in several nuclei. The right figure shows a variation of this process than can only happen if neutrinos are Majorana (and hence act as their own anti-particle). In this case, the final state anti-neutrinos can now be a closed loop - the neutrino can be emitted by one neutron and absorbed by the other. In this case, there are no neutrinos emitted. This has a profound effect on the outgoing electron energy spectrum, as shown below. The two neutrino double beta decay has a broad summed energy spectrum for the outgoing electrons because the neutrinos carry away a significant and variable fraction of the interaction energy. By contrast, in neutrinoless double beta decay the electrons take away all the available energy except for a small amount that goes into nuclear recoil. This is shown as a small peak near the enedpoint energy of the decay. Looking for this small peak at the endpoint is the way SNO+ will look for evidence for the Majorana nature of the neutrino. The plot below shows the expected measured spectrum in SNO+ for neutrinoless double beta decay of 130-Te loaded into the liquid scintillator, assuming the effective mass of the neutrino is 100 meV. Also shown are backgrounds from radioactive contaminants, solar neutrinos, and spill over of two neutrino double beta decay events. We hope to be running in this mode by early 2019.
SNO+ as a Media Phenomenon!
We have our own craft brew!
...and our own Recruiting Video!
...and Spiderman is a SNO+ Collaborator!
References: